Introduction to Battery Charge Circuits
A battery charge circuit is an essential component in any electronic device that relies on rechargeable batteries. It ensures that the batteries are charged safely and efficiently, prolonging their lifespan and maintaining optimal performance. In this article, we will explore the intricacies of battery charge circuits and discuss five customized functions that enable perfect charging.
What is a Battery Charge Circuit?
A battery charge circuit is an electronic circuit designed to control the charging process of rechargeable batteries. It regulates the voltage and current supplied to the battery, preventing overcharging, undercharging, and other detrimental conditions that can damage the battery or compromise its performance.
The primary components of a battery charge circuit include:
-
Power source: The power source provides the necessary energy to charge the battery. It can be an AC adapter, a USB port, or any other suitable power supply.
-
Voltage regulator: The voltage regulator ensures that the voltage supplied to the battery remains constant and within the specified range. It prevents overvoltage, which can damage the battery.
-
Current limiter: The current limiter restricts the amount of current flowing into the battery, preventing overcurrent conditions that can cause overheating and battery degradation.
-
Charge controller: The charge controller monitors the battery’s state of charge (SOC) and adjusts the charging process accordingly. It employs various charging algorithms, such as constant current (CC), constant voltage (CV), or a combination of both (CC-CV).
-
Protection circuitry: The protection circuitry safeguards the battery and the charging circuit from abnormal conditions, such as short circuits, reverse polarity, and overtemperature.
Importance of Battery Charge Circuits
Battery charge circuits play a crucial role in ensuring the longevity and reliability of rechargeable batteries. Some of the key reasons why battery charge circuits are important include:
-
Battery safety: Overcharging or exposing batteries to excessive current can lead to thermal runaway, causing the battery to swell, leak, or even explode. Battery charge circuits prevent these hazardous conditions by regulating the charging process.
-
Battery lifespan: Proper charging is essential for maximizing the number of charge cycles a battery can undergo before its capacity diminishes. Battery charge circuits employ optimized charging algorithms that minimize stress on the battery, extending its lifespan.
-
Device performance: Efficient battery charging ensures that devices can operate at their peak performance for longer periods. Battery charge circuits maintain the battery’s state of charge within the optimal range, providing a reliable power source for the device.
-
Cost savings: Replacing batteries prematurely due to improper charging can be costly. By incorporating battery charge circuits, manufacturers can reduce warranty claims and customer dissatisfaction associated with battery-related issues.
5 Customized Functions for Perfect Charging
Now that we understand the basics of battery charge circuits let’s explore five customized functions that enable perfect charging.
1. Multi-Stage Charging
Multi-stage charging is a charging technique that employs multiple charging stages to optimize the charging process. It typically consists of three main stages: bulk, absorption, and float.
-
Bulk stage: During the bulk stage, the battery is charged with a constant current (CC) until it reaches approximately 80% of its capacity. This stage replenishes the majority of the battery’s charge quickly.
-
Absorption stage: Once the battery reaches the absorption voltage threshold, the charging current gradually decreases while the voltage remains constant. This stage tops off the remaining 20% of the battery’s capacity and helps to equalize the charge across the individual cells.
-
Float stage: After the absorption stage, the charging voltage is lowered to the float voltage level. This stage maintains the battery at a full charge without causing overcharging. It compensates for the battery’s self-discharge and keeps it ready for use.
Multi-stage charging offers several benefits:
- It minimizes the stress on the battery by applying the appropriate charging current and voltage at each stage.
- It helps to balance the charge across individual cells, preventing overcharge and undercharge conditions.
- It maintains the battery at a full charge without causing damage due to overcharging.
2. Temperature Compensation
Temperature plays a significant role in battery charging. Charging at extreme temperatures (too hot or too cold) can severely impact the battery’s performance and lifespan. Temperature compensation is a feature in battery charge circuits that adjusts the charging voltage based on the battery’s temperature.
Temperature Range | Voltage Adjustment |
---|---|
< 0°C | -20 mV/°C |
0°C to 25°C | No adjustment |
> 25°C | -5 mV/°C |
Temperature compensation works by monitoring the battery’s temperature using a temperature sensor. The charging voltage is then adjusted according to the temperature coefficient of the battery chemistry. For example, lead-acid batteries typically require a voltage adjustment of -20 mV/°C for temperatures below 0°C and -5 mV/°C for temperatures above 25°C.
By compensating for temperature variations, battery charge circuits ensure that the battery is charged at the optimal voltage, preventing overcharge at high temperatures and undercharge at low temperatures. This function extends the battery’s lifespan and maintains its performance across a wide temperature range.
3. Charge Termination
Charge termination is a critical function in battery charge circuits that prevents overcharging. Overcharging occurs when the battery is continuously charged even after reaching its full capacity. It can lead to gas formation, heat generation, and other detrimental effects that shorten the battery’s lifespan.
Battery charge circuits employ various charge termination methods, depending on the battery chemistry and application requirements. Some common charge termination techniques include:
-
Voltage-based termination: The charging process is terminated when the battery voltage reaches a predefined threshold. This method is simple but may not account for the battery’s state of charge accurately.
-
Current-based termination: The charging process is terminated when the charging current drops below a certain threshold, indicating that the battery is fully charged. This method is more accurate than voltage-based termination but requires precise current sensing.
-
Timer-based termination: The charging process is terminated after a predetermined time, regardless of the battery’s state of charge. This method is used as a safety backup to prevent overcharging if other termination methods fail.
-
Coulomb counting: This method involves tracking the amount of charge entering and leaving the battery. The charging process is terminated when the net charge reaches the battery’s capacity. Coulomb counting provides accurate charge termination but requires additional circuitry for current measurement.
Implementing appropriate charge termination techniques ensures that the battery is not overcharged, prolonging its lifespan and preventing safety hazards.
4. Charge Equalization
Charge equalization is a function that balances the charge across individual cells in a multi-cell battery pack. In multi-cell configurations, such as series-connected cells, slight variations in cell capacities and internal resistances can lead to uneven charge distribution. Over time, this imbalance can cause some cells to be overcharged while others are undercharged, reducing the overall battery performance and lifespan.
Battery charge circuits with charge equalization capabilities actively monitor the voltage of each cell and apply corrective measures to maintain balance. There are two main methods for charge equalization:
-
Passive equalization: In passive equalization, the charge circuit uses resistors or other dissipative elements to discharge the cells with higher voltages until they match the voltage of the lower cells. This method is simple and cost-effective but can be inefficient and generate heat.
-
Active equalization: Active equalization involves redistributing the charge from higher-voltage cells to lower-voltage cells using switched capacitors or inductors. This method is more efficient than passive equalization but requires additional circuitry and control mechanisms.
Charge equalization ensures that all cells in a multi-cell battery pack are at the same state of charge, maximizing the battery’s capacity and preventing individual cell overcharge or undercharge conditions.
5. State of Charge (SOC) Monitoring
State of charge (SOC) monitoring is a function that estimates the remaining capacity of a battery as a percentage of its full capacity. Accurate SOC estimation is crucial for several reasons:
- It provides users with an indication of the remaining battery runtime.
- It enables devices to manage power consumption based on the available battery capacity.
- It helps to optimize the charging process by adapting the charging current and voltage based on the battery’s SOC.
Battery charge circuits employ various methods to estimate the SOC, including:
-
Voltage-based estimation: This method relies on the relationship between the battery’s voltage and its SOC. However, the voltage-SOC relationship is not always linear and can be affected by factors such as temperature and aging.
-
Coulomb counting: By measuring the current flowing into and out of the battery and integrating it over time, the charge circuit can estimate the change in the battery’s capacity. This method provides accurate SOC estimation but requires precise current measurement and calibration.
-
Kalman filtering: Kalman filtering is an advanced technique that combines voltage-based estimation and Coulomb counting to provide a more accurate SOC estimate. It uses a mathematical model of the battery and real-time measurements to update the SOC estimate continuously.
Implementing SOC monitoring in battery charge circuits enables users to make informed decisions about battery usage and helps to optimize the charging process for better battery performance and lifespan.
Frequently Asked Questions (FAQ)
- What is the difference between constant current (CC) and constant voltage (CV) charging?
-
Constant current charging involves applying a fixed charging current to the battery until it reaches a certain voltage threshold. Constant voltage charging, on the other hand, maintains a constant voltage across the battery while the charging current gradually decreases as the battery approaches full charge. CC charging is typically used in the bulk stage, while CV charging is used in the absorption and float stages.
-
Can I charge a lithium-ion battery with a lead-acid battery charger?
-
No, it is not recommended to charge a lithium-ion battery with a lead-acid battery charger. Lithium-ion batteries require specific charging algorithms and voltage/current profiles that differ from lead-acid batteries. Using an incompatible charger can damage the lithium-ion battery and pose safety risks.
-
What is the purpose of the protection circuitry in a battery charge circuit?
-
The protection circuitry in a battery charge circuit serves to safeguard the battery and the charging circuit from abnormal conditions. It includes features such as overvoltage protection, overcurrent protection, short-circuit protection, and reverse polarity protection. These protective measures prevent damage to the battery and ensure safe charging operations.
-
How does temperature affect battery charging?
-
Temperature has a significant impact on battery charging. Charging at high temperatures can cause the battery to degrade faster and may lead to safety issues such as thermal runaway. Charging at low temperatures can slow down the charging process and reduce the battery’s capacity. Battery charge circuits with temperature compensation adjust the charging voltage based on the battery’s temperature to ensure optimal charging performance.
-
What is the role of charge equalization in multi-cell battery packs?
- Charge equalization is essential in multi-cell battery packs to maintain balance among the individual cells. Due to manufacturing variations and differing cell aging rates, some cells may have slightly higher or lower capacities than others. Without charge equalization, these imbalances can lead to overcharging or undercharging of individual cells, reducing the overall battery performance and lifespan. Charge equalization techniques, such as passive or active balancing, ensure that all cells are at the same state of charge, maximizing the battery pack’s capacity and longevity.
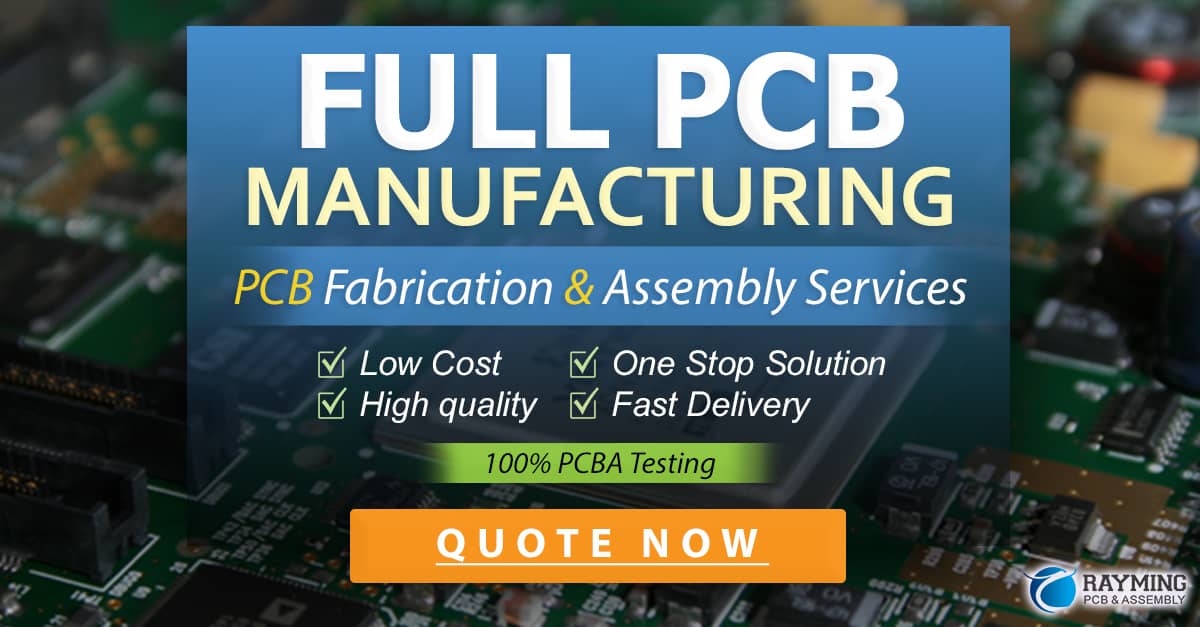
Conclusion
Battery charge circuits are vital components in ensuring the safe, efficient, and long-lasting operation of rechargeable batteries. By implementing customized functions such as multi-stage charging, temperature compensation, charge termination, charge equalization, and state of charge monitoring, battery charge circuits can optimize the charging process and maintain battery health.
As battery technologies continue to advance, so do the capabilities of battery charge circuits. Manufacturers and designers are constantly developing new techniques and algorithms to enhance charging performance, prolong battery lifespan, and ensure user safety.
Whether you are an electronic engineer designing a new product or a consumer looking to maximize the performance of your rechargeable devices, understanding the intricacies of battery charge circuits is crucial. By leveraging the customized functions discussed in this article, you can ensure that your batteries are charged perfectly, providing reliable and long-lasting power for all your electronic needs.
0 Comments