Introduction to PCB Crosstalk
In the realm of high-speed printed circuit board (PCB) design, crosstalk has emerged as a critical issue that demands meticulous attention and effective mitigation strategies. As digital systems continue to push the boundaries of speed and complexity, the phenomenon of crosstalk becomes increasingly pronounced, posing significant challenges to signal integrity and overall system performance. This article delves into the intricacies of PCB crosstalk, exploring its underlying causes, detrimental effects, and the various techniques employed to analyze and control its impact on high-speed PCB Designs.
What is PCB Crosstalk?
PCB crosstalk refers to the unwanted coupling of signals between adjacent traces or conductors on a printed circuit board. When a signal propagates through a trace, it generates electromagnetic fields that can interfere with nearby traces, inducing undesired voltage or current fluctuations. This interference can lead to signal distortion, timing issues, and even false logic transitions, compromising the integrity of the transmitted data.
Types of PCB Crosstalk
PCB crosstalk can manifest in two primary forms:
-
Inductive Crosstalk: Inductive crosstalk occurs when the magnetic field generated by a signal-carrying trace induces a voltage in a neighboring trace. This type of crosstalk is more prominent in traces with high current levels and longer parallel runs.
-
Capacitive Crosstalk: Capacitive crosstalk arises from the electric field coupling between adjacent traces. The voltage difference between the traces creates a parasitic capacitance, resulting in the transfer of charge and subsequent signal distortion.
Factors Influencing PCB Crosstalk
Several factors contribute to the severity of PCB crosstalk, including:
Trace Spacing and Geometry
The proximity of traces plays a crucial role in determining the extent of crosstalk. As the spacing between traces decreases, the likelihood and intensity of crosstalk increase. Additionally, the geometry of the traces, such as their width, thickness, and length, influences the coupling capacitance and inductance, thereby affecting crosstalk levels.
Signal Characteristics
The characteristics of the signals propagating through the traces also impact crosstalk. High-frequency signals with fast rise and fall times are more susceptible to crosstalk due to their broader frequency spectrum and increased coupling effects. Moreover, signals with larger voltage swings or higher current levels can induce stronger electromagnetic fields, exacerbating crosstalk.
Dielectric Properties
The properties of the dielectric material used in the PCB, such as its permittivity and Loss Tangent, influence the capacitive coupling between traces. Materials with higher permittivity values tend to increase the coupling capacitance, leading to more pronounced crosstalk effects.
Analyzing PCB Crosstalk
To effectively mitigate crosstalk in high-speed PCB designs, it is essential to employ appropriate analysis techniques. These techniques enable designers to predict and quantify crosstalk levels, identify problematic areas, and make informed design decisions. Some commonly used methods for analyzing PCB crosstalk include:
Electromagnetic Simulation
Electromagnetic (EM) simulation tools, such as 3D field solvers, allow designers to model and simulate the electromagnetic behavior of PCB Traces. These tools provide detailed insights into crosstalk levels, field distributions, and coupling effects, enabling designers to optimize trace routing and spacing for minimal crosstalk.
SPICE Simulation
SPICE (Simulation Program with Integrated Circuit Emphasis) is a widely used circuit simulation tool that can be employed to analyze crosstalk in PCB designs. By creating equivalent circuit models of the PCB traces and their coupling capacitances and inductances, designers can simulate the crosstalk behavior and assess its impact on signal integrity.
Worst-Case Analysis
Worst-case analysis involves identifying and simulating the most critical scenarios that can lead to maximum crosstalk. By considering factors such as trace alignment, signal timing, and simultaneous switching, designers can determine the worst-case crosstalk levels and ensure that the design remains robust under these conditions.
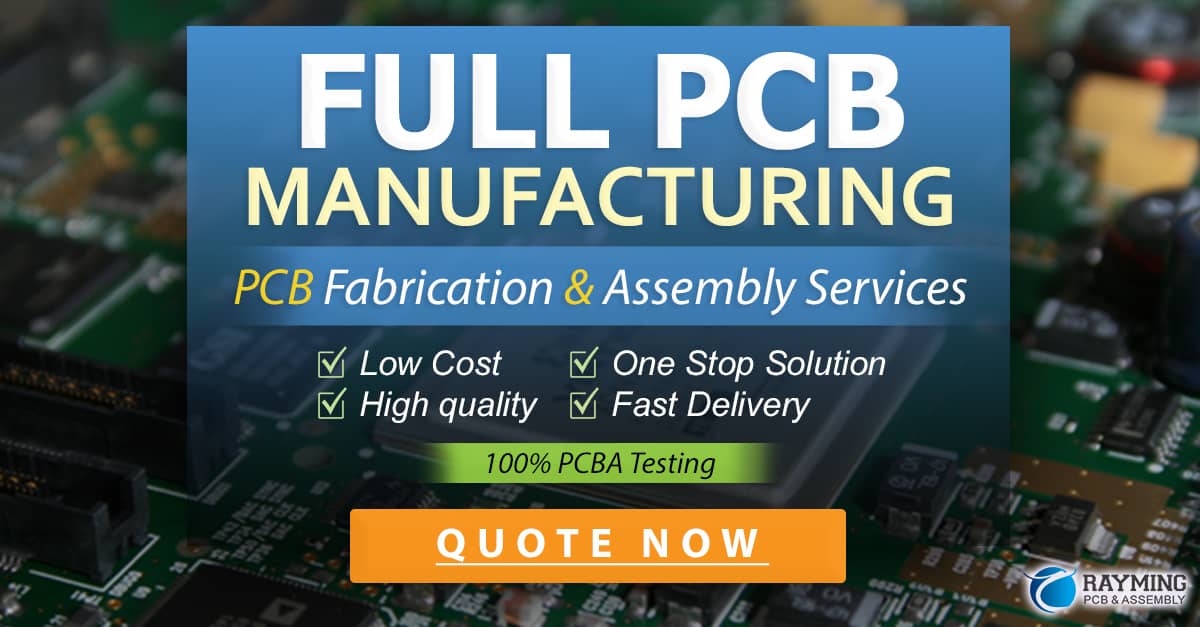
Controlling PCB Crosstalk
To minimize the adverse effects of crosstalk in high-speed PCB designs, several control techniques can be implemented. These techniques aim to reduce coupling, improve signal integrity, and maintain reliable system performance. Some effective crosstalk control strategies include:
Trace Spacing and Routing
Increasing the spacing between traces is one of the most straightforward methods to reduce crosstalk. By maintaining adequate separation between signal traces, especially those carrying high-speed or sensitive signals, designers can minimize the coupling capacitance and inductance, thereby reducing crosstalk levels. Additionally, careful routing techniques, such as avoiding long parallel runs and minimizing trace crossovers, can further mitigate crosstalk.
Shielding and Guarding
Shielding involves the use of conductive barriers, such as grounded traces or planes, to isolate sensitive signals from crosstalk-inducing traces. By placing grounded traces or planes adjacent to the signal traces, the electromagnetic fields are contained, reducing the coupling effects. Guarding, on the other hand, involves surrounding sensitive traces with grounded guard traces, creating a protective shield that minimizes crosstalk.
Differential Signaling
Employing differential signaling techniques can significantly reduce the impact of crosstalk in high-speed PCB designs. Differential signaling uses a pair of complementary signals, where the desired information is represented by the voltage difference between the two signals. This approach offers excellent noise immunity and cancels out common-mode interference, including crosstalk, as the induced noise appears equally on both signal lines.
Termination and Impedance Matching
Proper termination and impedance matching techniques help mitigate crosstalk by reducing signal reflections and ensuring clean signal transitions. By matching the impedance of the signal traces to the source and load impedances, designers can minimize signal overshoots, undershoots, and ringing, which can contribute to crosstalk. Techniques such as series termination, parallel termination, and differential termination can be employed based on the specific design requirements.
Signal Integrity Simulation and Analysis
Incorporating signal integrity simulation and analysis into the PCB-Design/”>PCB design process is crucial for identifying and addressing crosstalk issues early in the development cycle. Signal integrity tools allow designers to simulate the behavior of signals in the presence of crosstalk, assess the impact on timing and voltage margins, and optimize the design for robust performance. These tools provide valuable insights into signal quality, helping designers make informed decisions regarding trace routing, termination, and other crosstalk mitigation strategies.
FAQs
-
Q: What is the difference between inductive and capacitive crosstalk?
A: Inductive crosstalk occurs when the magnetic field generated by a signal-carrying trace induces a voltage in a neighboring trace, while capacitive crosstalk arises from the electric field coupling between adjacent traces due to the voltage difference between them. -
Q: How does trace spacing affect PCB crosstalk?
A: The spacing between traces directly influences the severity of crosstalk. As the spacing decreases, the coupling capacitance and inductance increase, leading to more pronounced crosstalk effects. Increasing the trace spacing is an effective way to reduce crosstalk in PCB designs. -
Q: What is the role of electromagnetic simulation in analyzing PCB crosstalk?
A: Electromagnetic simulation tools, such as 3D field solvers, enable designers to model and simulate the electromagnetic behavior of PCB traces. These tools provide detailed insights into crosstalk levels, field distributions, and coupling effects, allowing designers to optimize trace routing and spacing for minimal crosstalk. -
Q: How can differential signaling help mitigate crosstalk in high-speed PCB designs?
A: Differential signaling uses a pair of complementary signals, where the desired information is represented by the voltage difference between the two signals. This approach offers excellent noise immunity and cancels out common-mode interference, including crosstalk, as the induced noise appears equally on both signal lines, effectively reducing its impact on signal integrity. -
Q: Why is signal integrity simulation and analysis important for addressing crosstalk issues?
A: Signal integrity simulation and analysis tools allow designers to simulate the behavior of signals in the presence of crosstalk, assess the impact on timing and voltage margins, and optimize the design for robust performance. By incorporating these tools into the PCB design process, designers can identify and address crosstalk issues early in the development cycle, ensuring reliable system performance.
Conclusion
Crosstalk in high-speed PCB designs poses significant challenges to signal integrity and overall system performance. As digital systems continue to push the boundaries of speed and complexity, understanding and effectively mitigating crosstalk becomes increasingly critical. By analyzing the factors that influence crosstalk, such as trace spacing, signal characteristics, and dielectric properties, designers can employ various techniques to control its impact.
Through the use of electromagnetic simulation, SPICE simulation, and worst-case analysis, designers can predict and quantify crosstalk levels, identify problematic areas, and make informed design decisions. Implementing control strategies, such as optimizing trace spacing and routing, employing shielding and guarding techniques, utilizing differential signaling, and ensuring proper termination and impedance matching, can significantly reduce crosstalk and improve signal integrity.
Furthermore, incorporating signal integrity simulation and analysis into the PCB design process enables designers to identify and address crosstalk issues early in the development cycle, saving time and resources while ensuring robust system performance.
By understanding the intricacies of PCB crosstalk and adopting effective analysis and control techniques, designers can overcome the challenges posed by crosstalk in high-speed PCB designs. This knowledge empowers them to create reliable, high-performance digital systems that can withstand the ever-increasing demands of modern electronics.
0 Comments