Understanding the Concept of Glass Transition Temperature
The Amorphous State
To comprehend the concept of glass transition temperature, it is essential to understand the amorphous state of materials. Amorphous materials lack the long-range order found in crystalline structures. Instead, they possess a random arrangement of molecules or atoms. This lack of order gives amorphous materials unique properties, such as transparency and isotropic behavior.
The Glassy and Rubbery States
Amorphous materials can exist in two distinct states: the glassy state and the rubbery state. In the glassy state, the material is rigid, brittle, and has a limited ability to deform under stress. The molecules or atoms in this state have limited mobility and are essentially “frozen” in place. Conversely, in the rubbery state, the material is soft, flexible, and can easily deform under stress. The molecules or atoms have increased mobility and can move relative to one another.
The Glass Transition Temperature Range
The glass transition temperature is not a single, well-defined point but rather a temperature range over which the material undergoes the transition from the glassy to the rubbery state. This transition occurs gradually as the temperature increases, with the material’s properties changing continuously throughout the range.
The glass transition temperature range is typically characterized by a significant change in the material’s heat capacity, thermal expansion coefficient, and mechanical properties, such as elastic modulus and viscosity.
Factors Affecting Glass Transition Temperature
Several factors can influence the glass transition temperature of a material, including:
Molecular Structure
The molecular structure of a material plays a crucial role in determining its glass transition temperature. Factors such as chain length, branching, cross-linking, and the presence of functional groups can all affect the Tg. Generally, materials with longer chains, higher degrees of branching, and cross-linking tend to have higher glass transition temperatures due to the increased interactions between molecules and the reduced mobility of the chains.
Molecular Weight
The molecular weight of a polymer can also influence its glass transition temperature. As the molecular weight increases, the Tg tends to increase as well. This is because longer chains have more entanglements and interactions, which restrict their mobility and require more energy (i.e., higher temperatures) to overcome.
Plasticizers
Plasticizers are additives that can be incorporated into a material to lower its glass transition temperature. These molecules typically have a smaller size and lower molecular weight compared to the main polymer chains. They work by increasing the free volume between the chains, allowing for greater molecular mobility at lower temperatures. Common plasticizers include phthalates, adipates, and citrates.
Copolymerization
Copolymerization involves the incorporation of two or more different monomers into a single polymer chain. By carefully selecting the monomers and their relative proportions, it is possible to tune the glass transition temperature of the resulting copolymer. This approach is often used to create materials with specific properties tailored to a particular application.
Measuring Glass Transition Temperature
There are several techniques available for measuring the glass transition temperature of a material, each with its own advantages and limitations. Some of the most common methods include:
Differential Scanning Calorimetry (DSC)
Differential scanning calorimetry is a widely used technique for determining the glass transition temperature of a material. In this method, a small sample of the material is placed in a crucible and heated alongside a reference crucible containing an inert material. The difference in heat flow between the sample and reference is measured as a function of temperature. The glass transition is observed as a step change in the heat flow signal, indicating a change in the material’s heat capacity.
Advantages of DSC | Limitations of DSC |
---|---|
Small sample size required (1-10 mg) | Limited to materials that do not undergo decomposition or other transitions in the temperature range of interest |
Rapid analysis time (typically < 1 hour) | May not detect weak or broad transitions |
Provides information on other thermal events (e.g., melting, crystallization) | Requires careful sample preparation and calibration |
Dynamic Mechanical Analysis (DMA)
Dynamic mechanical analysis is another common technique for measuring the glass transition temperature of a material. In this method, a small sample of the material is subjected to a sinusoidal stress or strain, and the resulting deformation is measured as a function of temperature. The glass transition is observed as a peak in the loss modulus (E”) and a step change in the storage modulus (E’).
Advantages of DMA | Limitations of DMA |
---|---|
Provides information on mechanical properties (e.g., modulus, damping) | Requires larger sample sizes compared to DSC (typically 10-50 mm in length) |
Can measure transitions that are weak or broad | May be affected by sample geometry and clamping effects |
Allows for the study of frequency-dependent behavior | Limited to materials that can be formed into suitable geometries (e.g., films, fibers, bars) |
Thermomechanical Analysis (TMA)
Thermomechanical analysis is a technique that measures the dimensional changes of a material as a function of temperature. The glass transition is observed as a change in the slope of the dimensional change curve, indicating a change in the material’s coefficient of thermal expansion.
Advantages of TMA | Limitations of TMA |
---|---|
Provides information on dimensional stability and thermal expansion | Limited to materials that do not undergo significant softening or flow in the temperature range of interest |
Can measure transitions that are weak or broad | Requires larger sample sizes compared to DSC (typically 5-10 mm in length) |
Allows for the study of anisotropic behavior | May be affected by sample geometry and contact effects |
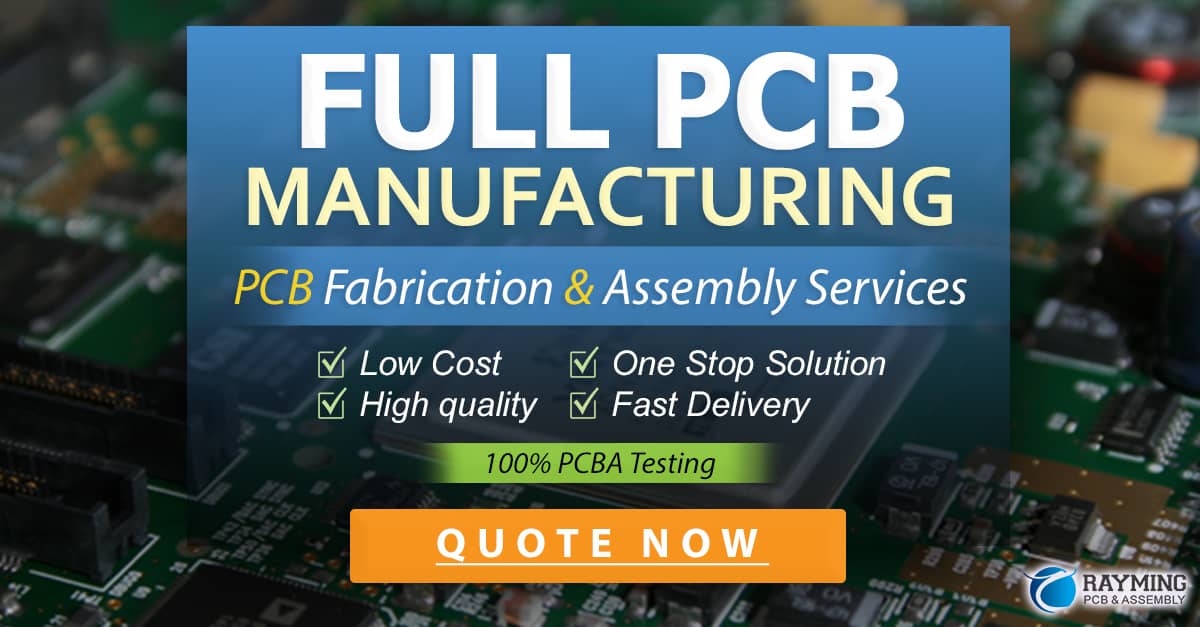
Applications of Glass Transition Temperature
Understanding and controlling the glass transition temperature of a material is crucial for many applications, as it determines the temperature range over which the material can be effectively used. Some examples include:
Polymer Processing
In polymer processing techniques such as injection molding, extrusion, and blow molding, the glass transition temperature plays a critical role in determining the processing conditions. The material must be heated above its Tg to allow for sufficient molecular mobility and flow during the shaping process. However, if the temperature is too high, the material may undergo degradation or other undesirable changes.
Packaging Materials
Many packaging materials, such as plastic films and containers, rely on the glass transition temperature to maintain their structural integrity and barrier properties. For example, in food packaging, it is essential to select materials with a Tg well above the expected storage and use temperatures to prevent softening, deformation, or loss of barrier properties.
Composite Materials
In composite materials, the glass transition temperature of the polymer matrix plays a crucial role in determining the overall performance of the composite. The Tg of the matrix should be well above the expected service temperature to ensure that the composite maintains its mechanical properties and dimensional stability. Additionally, the Tg of the matrix can influence the interfacial adhesion between the matrix and reinforcing fibers, affecting the stress transfer and overall composite strength.
Biomedical Applications
In biomedical applications, such as drug delivery systems and tissue engineering scaffolds, the glass transition temperature of the polymeric materials used is of utmost importance. For drug delivery systems, the Tg can be tuned to control the release kinetics of the encapsulated drug, allowing for sustained or targeted delivery. In tissue engineering, the Tg of the scaffold material can influence cell attachment, proliferation, and differentiation, as well as the mechanical properties of the scaffold.
Frequently Asked Questions (FAQ)
-
Q: What is the difference between glass transition temperature and melting temperature?
A: The glass transition temperature (Tg) is the temperature range over which an amorphous material transitions from a hard, glassy state to a soft, rubbery state. In contrast, the melting temperature (Tm) is the temperature at which a crystalline material transitions from a solid to a liquid state. Amorphous materials do not have a true melting point, as they do not possess the long-range order found in crystalline structures. -
Q: Can a material have more than one glass transition temperature?
A: Yes, some materials, particularly copolymers and blends, can exhibit multiple glass transition temperatures. This occurs when the different components of the material have distinct Tg values, resulting in separate transitions for each phase. The presence of multiple Tg values can be used to tailor the properties of the material for specific applications. -
Q: How does the addition of fillers or reinforcements affect the glass transition temperature of a polymer?
A: The effect of fillers or reinforcements on the glass transition temperature of a polymer depends on several factors, such as the type and concentration of the additive, as well as the interactions between the additive and the polymer matrix. In some cases, the addition of fillers or reinforcements can increase the Tg by restricting the mobility of the polymer chains. In other cases, the additives may have a plasticizing effect, lowering the Tg of the material. -
Q: Can the glass transition temperature of a material change over time?
A: Yes, the glass transition temperature of a material can change over time due to various factors, such as aging, exposure to environmental conditions (e.g., moisture, UV radiation), and chemical changes (e.g., oxidation, hydrolysis). These changes can lead to a gradual alteration of the material’s properties, including its Tg. It is essential to consider the long-term stability of a material’s Tg when designing products for extended use. -
Q: How can I modify the glass transition temperature of a polymer?
A: There are several ways to modify the glass transition temperature of a polymer, depending on the desired outcome and the specific application. Some common methods include: - Copolymerization: Incorporating different monomers into the polymer chain can alter the Tg, depending on the properties of the monomers used.
- Plasticization: Adding plasticizers to the polymer can lower its Tg by increasing the free volume between the chains and enhancing molecular mobility.
- Cross-linking: Increasing the degree of cross-linking in a polymer can raise its Tg by restricting the mobility of the chains and increasing the energy required for the transition.
- Molecular weight modification: Changing the molecular weight of the polymer can influence its Tg, with higher molecular weights generally resulting in higher Tg values.
In conclusion, the glass transition temperature is a critical parameter in material science, particularly for polymers and amorphous materials. It determines the temperature range over which a material transitions from a hard, glassy state to a soft, rubbery state, and has significant implications for the material’s mechanical, thermal, and electrical properties. By understanding the factors that influence Tg and the methods available for measuring and modifying it, engineers and scientists can design and manufacture products with optimized performance for a wide range of applications.
0 Comments